The Battle of Light: From Waves to Particles Explained
Written on
Chapter 1: Understanding Photons
The photon is a fundamental particle representing the smallest unit of light and various forms of electromagnetic radiation. These energy packets can appear in different colors or even manifest as X-rays or gamma rays, depending on their energy levels.
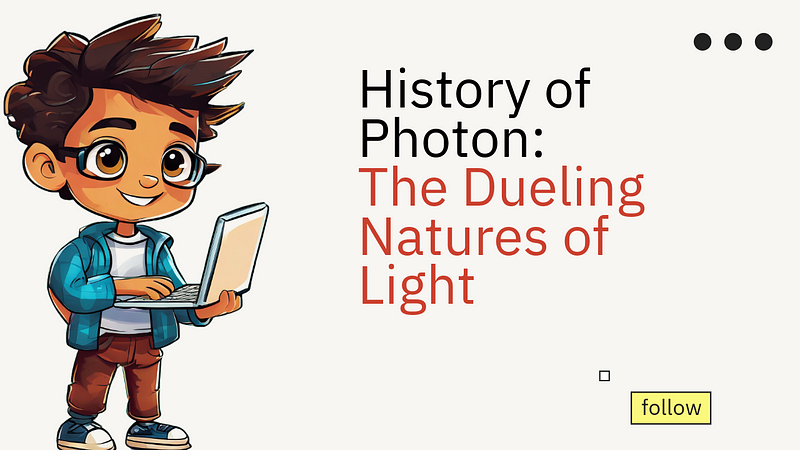
The narrative of the photon is an intriguing account of the conflict between two seemingly opposing concepts: light as a wave versus light as a particle. While photons are relatively modern discoveries, pinpointing their origins and the timeline is complex.
The journey of the photon began with Max Planck's groundbreaking findings in 1900. Planck sought to address the notorious 'ultraviolet catastrophe.' Envision a perfect absorber and emitter of light, a theoretical construct known as a black body. As its temperature rises, it emits an increasing variety of colors. Classical physics predicted the emission of light from a black body through the Rayleigh-Jeans Law, which suggested that shorter wavelengths would lead to an infinite increase in emitted energy. However, this did not align with real-world observations, as hot objects do not emit infinite brightness in the ultraviolet range.
To resolve this paradox, Planck proposed that electromagnetic radiation is quantized, existing in discrete energy packets. Although he did not fully understand the rationale behind this quantization, he believed it was linked to the emission process.
In 1905, Einstein expanded upon Planck's idea, asserting that quantization is an intrinsic characteristic of electromagnetic radiation unrelated to the emission process. He utilized this concept to elucidate the photoelectric effect.
The photoelectric effect describes how light can dislodge electrons from a metallic surface. This phenomenon serves as compelling evidence for light's particle-like behavior through energy packets called photons. The classical wave theory of light fails to explain several key observations:
Threshold Frequency: A minimum frequency (color) of light is required to release electrons. Regardless of how intense the light is, if its frequency falls below this threshold, no electrons will be ejected.
Kinetic Energy Dependence: The energy of the emitted electrons is contingent on the light's frequency, not its intensity. Higher frequency light results in electrons being ejected with greater kinetic energy, even if the light is dimmer.
How Photons Clarify These Points:
Einstein's photon theory reconciles these discrepancies:
Light as Energy Packets: Light acts not only as a wave but also as particles called photons. Each photon carries energy dictated by its frequency (higher frequency = higher energy).
Energy Transfer: When a photon collides with an electron in the metal, it transfers all its energy in one impact.
Threshold Explanation: If a photon’s energy (based on its frequency) is insufficient to overcome the binding energy of the electron, it cannot dislodge it. This accounts for the threshold frequency—only photons with energy exceeding a certain level can release electrons.
Intensity and Photon Count: The intensity of light determines the number of photons striking the metal, rather than the energy of each photon. Therefore, a brighter light leads to more electrons being ejected statistically (more photons impacting), yet each photon’s energy is still tied to its frequency.
Initially, many were skeptical of Einstein's photon theory regarding the photoelectric effect. His depiction of light as energy packets contradicted the long-established wave theory. The wave theory had successfully explained phenomena like reflection and refraction for centuries. The notion of quantized light energy was revolutionary, with Max Planck's earlier quantization concept regarded merely as a mathematical tool rather than a universal truth. Even distinguished physicists like Robert Millikan, who later confirmed the effect through experiments, initially rejected the photon concept.
In 1916, Millikan conducted photoelectric effect experiments and found that Einstein's equations aligned with the observed data. However, skepticism lingered.
It wasn't until Arthur Compton's 1923 experiment, which demonstrated X-rays scattering off electrons, that the debate was effectively settled. In this experiment, X-rays behaved like particles colliding with electrons, transferring momentum much like billiard balls. This observation reinforced the notion of light as particles (photons) carrying momentum.
The particle aspect of light offered a new lens through which to view electromagnetic interactions, warranting further exploration on its own.
You can support me on Kofi or by sharing this article.
If you enjoy free resources like I do, consider following me and subscribing to the newsletter. I will be sharing more about scholarships, fellowships, and data science topics. If you appreciated this article, please clap and share it. Until next time!
Chapter 2: The Impact of Einstein's Photon Theory
This video explores the philosophical implications of Einstein's question, "Is the moon there when no one looks?" and its relevance to the nature of reality.
David Albert discusses how Einstein's theories accurately describe the strange phenomena of quantum mechanics, emphasizing the legacy of the photon concept.